Press Releases
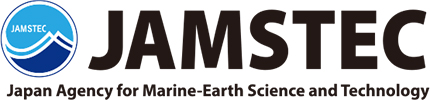
JAMSTEC
Physical Mechanisms Controlling Decadal Sea Ice Variability in the Weddell Sea
―Investigating the Fundamental Roles of the Weddell Gyre and Local Winds―
1. Key Points
- ◆
- Analysis of ice core-based paleoclimate data and sea ice satellite data indicates that sea ice cover in the Weddell Sea fluctuates on a 10-20-year scale.
- ◆
- Decadal sea ice variability in the Weddell Sea is associated with local surface wind and ocean temperature variations below the sea ice resulting from changes in the horizontal heat transport by the Weddell Gyre.
- ◆
- Accurate prediction of decadal sea ice variability in the Weddell Sea requires accurate representation of decadal variations in local winds and the Weddell Gyre in climate models.
2. Abstract
Drs. Yushi Morioka and Swadhin K. Behera (Application Laboratory, JAMSTEC) have identified that sea ice cover in the Weddell Sea (an Antarctic sea located south of the Atlantic Ocean) varies resulting from decadal variations in local winds and ocean circulation in the Weddell Sea, called the Weddell Gyre.
It is widely known that seasonal-interannual and decadal variations appear in the Antarctic sea ice records, and the latter has a periodicity of 10-20 years. However, decadal sea ice variability and underlying physical mechanisms have not been well studied. Although some studies have investigated decadal sea ice variations in the Pacific sector of the Antarctic seas (e.g., Ross and Amundsen seas), little is known about the underlying physical mechanisms in the Weddell Sea.
In this study, the analysis of ice core-based paleoclimate data, provided by NOAA/GFDL, and sea ice satellite data showed that sea ice cover in the Weddell Sea undergoes decadal variations, with a periodicity of 10-20 years. Furthermore, long-term sea ice simulations using the “Data Analyzer System” supercomputer suggest that decadal sea ice variability in the Weddell Sea is driven by local wind and ocean temperature variations below the sea ice, which are promoted by ocean circulation (the Weddell Gyre).
These results imply that accurate representation of local winds and ocean circulation in the Weddell Sea is necessary for accurately predicting decadal sea ice variability in this region. A greater number of in situ observations in the subsurface ocean below the sea ice are required to validate and improve the sea ice simulation and prediction in climate models.
The research paper will be published online on August 23 in the Journal of Geophysical Research: Oceans. This research used ice core-based paleoclimate data provided by NOAA/GFDL under the Memorandum of Understanding between NOAA and JAMSTEC, was supported by JSPS Kakenhi (JP19K14800).
- Application Laboratory, VAiG, JAMSTEC
3. Introduction
The Weddell Sea, an Antarctic sea located south of the Atlantic Ocean, is an important region where Antarctic Bottom Water formation occurs, regulating global, long-term climate through thermohaline circulation (※1). Sea ice cover in the Weddell Sea affects heat, freshwater, and gas exchanges between the atmosphere and the ocean. Since sea ice variability in the Weddell Sea influences water mass variations, understanding of sea ice variability is crucial.
Previous studies reported that sea ice cover in the Weddell Sea undergoes seasonal and interannual variations, but few studies have examined decadal sea ice variations in the Weddell Sea. For example, one study analyzed a 20-year satellite dataset of sea ice cover and reported that decadal sea ice variability in the Weddell Sea is caused by changes in sea ice transport by local winds. The direct role of local wind variations was also reported in ice-ocean simulation experiments.
However, previous studies have not investigated the role of ocean circulation changes in the Weddell Sea. Decadal variations in local ocean circulation, called the Weddell Gyre, may modulate ocean heat transport and affect ocean temperature below the sea ice. No studies to date have examined physical processes from the perspective of ice-ocean interactions.
In this study, an attempt is made to identify characteristics of decadal sea ice variability in the Weddell Sea from the analysis of ice core-based paleoclimate data and sea ice satellite data. Moreover, physical mechanisms underlying decadal sea ice variability in the Weddell Sea are investigated by conducting long-term sea ice simulations using a climate model.
4. Results
Ice core-based paleoclimate data suggest that sea ice varied on a decadal scale in the Weddell Sea (Figure 1). The spectrum analysis of surface air temperature over the past 2000 years, reconstructed using an ice core dataset at Berkner Island (Figure 1a), indicates that surface air temperature in the southern Weddell Sea has a decadal peak, with a periodicity of around 14 years (Figure 1b). This implies that sea ice cover in the southern Weddell Sea also undergoes decadal variations because it is closely associated with the surface air temperature in the Weddell Sea.
The standard deviation of the sea ice concentration (SIC), averaged over nine years, observed by satellites after 1982 (Figure 2a) shows that decadal sea ice variability in the northern Weddell Sea is higher than that in the southern Weddell Sea. A comparison among the SIC and atmospheric anomalies averaged from the northern Weddell Sea (Figure 2b) indicates that the high sea ice era (increased sea ice extent) is associated with weaker westerly winds (i.e., easterly wind anomalies), while the low sea ice era (decreased sea ice extent) is associated with stronger westerly winds (i.e., westerly wind anomalies). In general, weaker westerly winds weaken the northward Ekman current in the Weddell Sea, limiting the upwelling of warm water from the subsurface ocean, reducing ocean temperature below sea ice, and promoting sea ice formation. The anomalies of other variables such as meridional wind, wind stress curl, and net surface heat flux show weaker associations with SIC anomalies (Figure 2b).
A 300-year simulation was performed using the SINTEX-F2 (※2) climate model, on the “Data Analyzer System”, a JAMSTEC supercomputing system, to investigate physical mechanisms. The control (CTL) experiment accurately captures the periodicity and spatial pattern of decadal sea ice variability in the Weddell Sea (Figure not shown). The time series of the lag regressions of ocean temperature against the SIC averaged in the northern Weddell Sea (Figure 3a) shows that the ocean temperature at a depth of 200 m decreases anomalously, six years before the high sea ice era. Analysis of the lag regressions of ocean temperature tendency and its components in the upper 200 m (Figure 3b) shows that the negative trend of ocean temperature, occurring nine and six years before the high sea ice era, is mostly due to vertical ocean temperature advection and diffusion (i.e., the residual components of the heat budget). The time series of the lag regressions of vertical ocean velocity (Figure 3c) shows a weakening of ocean upwelling, nine and six years before the high sea ice era. Furthermore, the lag regressions of sea level pressure (SLP) and surface horizontal winds, nine years before the high sea ice era (Figure 3d), suggest a weakening of westerly winds in the Weddell Sea in association with the higher SLP in Antarctica and the lower SLP in the midlatitudes, representing a negative phase of the Southern Annular Mode (SAM; ※3). These results suggest that large-scale atmospheric variations in the Southern Hemisphere modulate local winds in the Weddell Sea and affect the upwelling of warm water from the subsurface ocean, and hence ocean temperature below the sea ice.
The role of local ice-ocean interaction is further investigated through another modeling experiment, called the SAOWED experiment, wherein the interannual sea surface temperature (SST) variations outside of the Weddell Sea and the South Atlantic are suppressed by monthly climatology of the SST from the CTL experiment. The SAOWED experiment simulated major characteristics of decadal sea ice variability in the Weddell Sea, as per the CTL experiment. The time series of the lag regressions of ocean temperature in the northern Weddell Sea (Figure 4a) shows that the ocean temperature below 200 m decreases anomalously, six years before the high sea ice era. Analysis of the ocean temperature tendency in the upper 200 m (Figure 4b) indicates that the negative tendency nine and six years before the high sea ice era is due to meridional ocean temperature advection. However, six and three years before the high sea ice era, the same tendency can be attributed to zonal ocean temperature advection. Furthermore, the lag regressions of ocean currents and density in the upper 200 m show a strengthening of clockwise ocean circulation, called the Weddell Gyre, with a higher density nine (Figure not shown) and six years (Figure 4c) before the high sea ice era. The lag regressions of ocean salinity in the upper 200 m (Figure 4d) indicates that increasing ocean salinity contributes the most to ocean density six years before the high sea ice era. The increased ocean salinity is promoted by increasing surface evaporation, resulting from decreasing sea ice extent six years before the high sea ice era. These results suggest that subject to decadal sea ice variability, the changes in the intensity of the Weddell Gyre modulate horizontal ocean heat transport and thus, ocean temperature below the sea ice.
5. Future Perspectives
This study identified characteristics of the decadal sea ice variability in the Weddell Sea from the analysis of ice core-based paleoclimate data and sea ice satellite data. Long-term sea ice simulations using a climate model identified two physical mechanisms (Figure 5): (i) local wind variations in the Weddell Sea modulate the upwelling of warm water from the subsurface ocean, affecting the ocean temperature below the sea ice (Figure 5a) and (ii) subject to the decadal sea ice variability, changes in the intensity of the Weddell Gyre modulate horizontal ocean heat transport, and thus ocean temperature below the sea ice (Figure 5b).
These results advance our understanding of the physical processes underlying decadal sea ice variability by providing accurate predictions. Accurate representation of the Weddell Gyre and local wind variations in climate models is necessary for the accurate prediction of decadal sea ice variability in the Weddell Sea. Since there are few ocean observations in the Weddell Sea, a greater number of in situ observations in the subsurface ocean below the sea ice are required for validating and improving the accuracy of sea ice simulation in climate models. Incorporating additional observational data into climate models for sea ice prediction in the Weddell Sea is the focus of our current research and will be reported in a subsequent study.
These research findings can also be applied for understanding decadal sea ice variability in other Antarctic and Arctic seas. Further in situ observations in these regions will help improve climate models, benefiting long-term sea ice prediction in the future.
【Supplementary Information】
- ※1
- Thermohaline circulation:
a global oceanic circulation regime that occurs over a few thousand years and facilitates the transportation of heat and nutrients in the ocean. One of the sources for the thermohaline circulation lies in the Weddell Sea where cold water with high salinity, called the Antarctic Bottom Water, forms and spreads into the other ocean basins.
- ※2
- SINTEX-F2:
ocean and atmosphere coupled general circulation model developed on the Earth Simulator under an international research collaboration between European research agencies and JAMSTEC. The model is written using a series of numerical program codes describing the physical and dynamical processes of the ocean and climate systems. The SINTEX-F2 model is mainly used for physical process and prediction studies on climate variability.
- ※3
- Southern Annular Mode (SAM):
it is known as the dominant atmospheric variability in the mid-high latitudes of the Southern Hemisphere. A positive phase of the SAM is associated with high-pressure anomalies at mid latitudes and low-pressure anomalies at high latitudes, leading to strengthening of the westerly winds at mid latitudes. A similar but contrasting process occurs during a negative phase of the SAM.
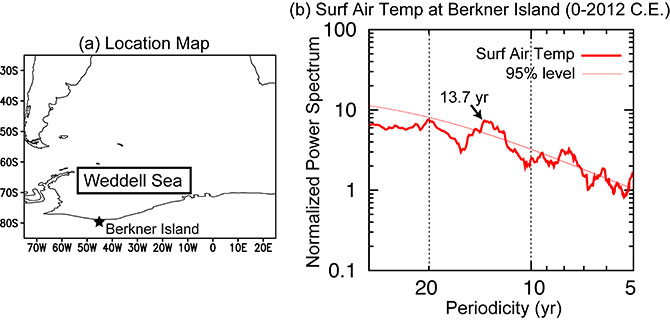
Figure 1: (a) Location of the Weddell Sea and Berkner Island (79.57°S, 45.72°W). (b) Power spectrum of the annual mean air temperature (thick red line) reconstructed using ice core data for the period of 0-2012 C.E.. The power spectrum is normalized by the time series variances. A thin red line shows the 95% confidence level.
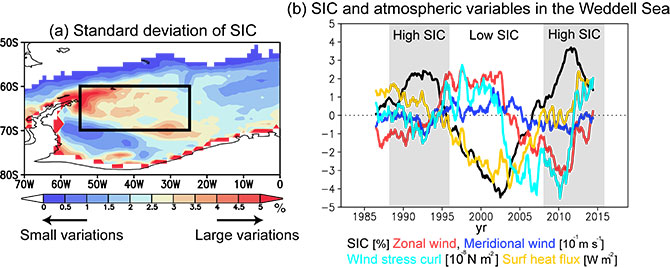
Figure 2: (a) Standard deviation of the nine-year mean sea ice concentration (SIC, in %). A black box shows a region of interest in the Weddell Sea (70-60°S, 55-25°W). We used monthly sea ice observation data during 1982-2018 from the OISSTv2 dataset. (b) Time series of the nine-year mean SIC (black line, in %), zonal wind (red line, in 10-1 m s-1), meridional wind (blue line, in 10-1 m s-1), wind stress curl (turquoise line, in 10-8 N m-2), and net surface heat flux (yellow line, in W m-2) anomalies averaged in the black box. We used atmospheric variables from the ERA5 reanalysis product.
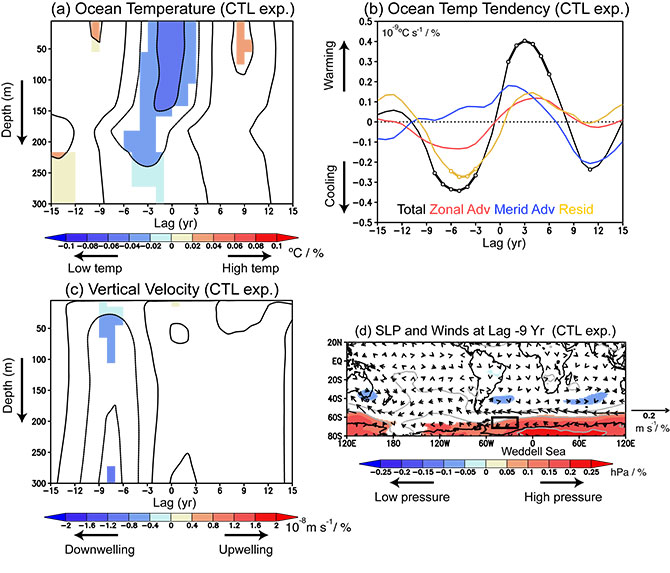
Figure 3: Results of the 300-year CTL experiment using the SINTEX-F2 model. (a) Lag regression of ocean temperature (in °C / %) in the Weddell Sea, against the SIC averaged in the black box of the Weddell Sea (see Fig. 3d). The x-axis shows lag years (in years, yr), while the y-axis indicates the depth (in meters, m). The Lag 0 year corresponds to the year with the maximum SIC increase in the Weddell Sea. (b) Lag regression of the ocean temperature tendency (black line; in 10-9 °C s-1 / %) in the upper 200 m and contributions from zonal ocean temperature advection (red line), meridional ocean temperature advection (blue line), and residuals (yellow line) including vertical ocean temperature advection and diffusion. (c) Lag regression of the vertical ocean current (in 10-8 m s-1 / %). (d) Lag regression of sea level pressure (SLP; in hPa / %) and horizontal winds (in m s-1 / %) at 10 m from the surface in Lag -9 years.
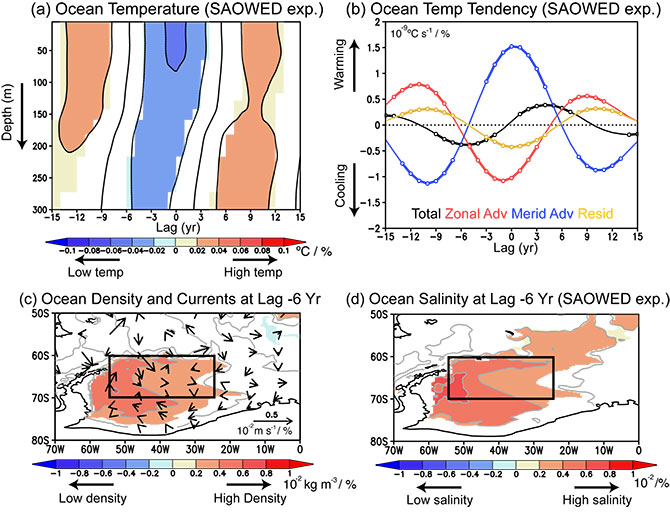
Figure 4: Results of the 300-year SAOWED experiment using the SINTEX-F2 model. (a) Lag regression of ocean temperature (in °C / %) in the Weddell Sea, against the SIC averaged in the black box of the Weddell Sea (see Fig. 4c). (b) Lag regression of the ocean temperature tendency (black line; in 10-9 °C s-1 / %) in the upper 200 m and contributions from zonal temperature advection (red line), meridional temperature advection (blue line), and residuals (yellow line) including vertical temperature advection and diffusion. (c) Lag regression of ocean density (in 10-2 kg m-3 / %) and currents (in 10-2 m s-1 / %) in the upper 200 m at Lag -6 years. (d) Lag regression of ocean salinity (10-2 / %) in the upper 200 m at Lag -6 years.
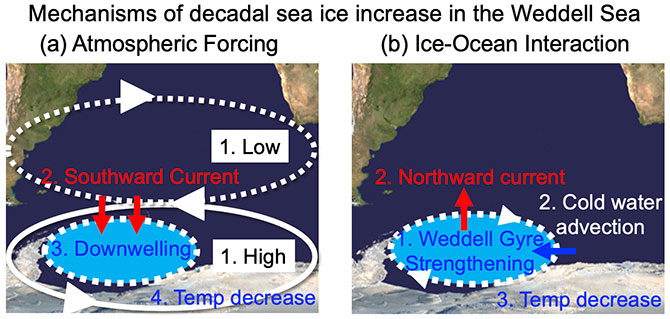
Figure 5: Two mechanisms accounting for increasing decadal sea ice extent in the Weddell Sea. (a) Role of atmospheric forcing. An anomalous high-pressure system in the Weddell Sea weakens the westerly winds (1), leading to the weakening of the northward current (i.e., anomalous southward current) in the northern part of the Weddell Sea (2). This prevents the upwelling of warm water (i.e., anomalous downwelling) from the subsurface ocean in the Weddell Sea (3). As a result, the ocean temperature in the upper ocean anomalously decreases (4), increasing sea ice extent in the Weddell Sea. (b) Role of local ice-ocean interaction. In response to decreasing sea ice extent in the Weddell Sea, the evaporation from the ocean surface enhances, generating highly saline water in the upper ocean. This increases ocean density and strengthens the clockwise ocean circulation, called the Weddell Gyre (1). The stronger Weddell Gyre transports warm water northward from the northern edge of the Weddell Sea and advects cold water westward from the eastern edge of the Weddell Sea (2). As a result, the ocean temperature in the upper ocean anomalously decreases, increasing sea ice extent in the Weddell Sea (3). Similar but contrasting mechanims for (a) and (b) are observed for decreasing decadal sea ice extent in the Weddell Sea.
Contacts
- (For this study)
- Yushi Morioka, Researcher, Research Institute for Value-Added-Information Generation (VAiG), Application Laboratory (APL), Climate Variability Prediction and Application Research Group, JAMSTEC
- (For press release)
- Public Relations Section, Marine Science and Technology Strategy Department, JAMSTEC